Overall objective: To determine the adaptations of HAB species and how they help to explain their proliferation or harmful effects.
Rationale. Each species has adaptive characteristics that define its niche, that is, the suite of physiological, morphological and life cycle features that determine its distribution in space and time. Within GEOHAB, specific characteristics and adaptations of HAB species were investigated in different marine ecosystem types, including upwelling systems (Pitcher et al. 2017), fjords and coastal embayments (Roy et al. 2017), stratified systems (Berdalet et al. 2017b) and eutrophic systems (Glibert and Burford 2017). Important contributions were provided for our understanding of i) the diversity of life histories of various HAB species – such as production and germination of resting stages, sexual reproduction – and their role in population dynamics (reviewed in Bravo and Figueroa 2014, Azanza et al. 2017); ii) the potential positive and negative interactions amongst species mediated by various allelochemical compounds (Ianora et al. 2011); iii) physiological adaptations of, for example, species producing long-lasting brown tides (Gobler and Sunda 2012); iv) complex interactions between HABs and their grazers, parasites and viruses (e.g., Chambouvet et al. 2008, Lu et al. 2016); v) in the case of non-swimming organisms (diatoms and cyanobacteria), ability to regulate buoyancy to access light and nutrients; and vi) genetic variability among cyanobacteria strains within populations and over time of a wide range of physiological adaptation strategies including akinete (=cyst) formation under adverse conditions, superior nutrient scavenging capacity, fixing atmospheric nitrogen, and synthesis of secondary metabolites (O’Neil et al. 2012). Finally, there is increasing evidences of the mixotrophic (combination of phototrophic with phagotrophic and/or osmotrophic nutritional modes) capacity of many HAB species, but their physiology is not known yet (Mitra and Flynn 2010).
Improvements in the characterization, both at theoretical and observational levels, of physical dynamics at the small scales of biological relevance have facilitated a better understanding of the physical-biological interactions that modulate HAB dynamics, for instance, in thin layers in stratified systems (e.g., GEOHAB 2008, GEOHAB 2013, Berdalet et al. 2014, Raine et al. in press). Stratification is a key factor modulating phytoplankton bloom dynamics and likely, there are also common strategies involved in the proliferation of cells in thin layers. However, the particular mechanisms leading to the selection of different noxious taxa such as Karenia, Dinophysis or Pseudo-nitzschia, are not known.
Detecting unique characteristics and adaptations of HAB species in particular environments could help in the development of predictive models. However, cosmopolitan rather than unique species thrive in upwelling systems (Smayda 2010) and no specific flora have been identified in fjords or coastal embayments (Roy et al. 2017). Even though some species may be specially favored by eutrophic conditions, the general view is that there is a wide range of combined nutrient availability and physical dynamics that define variable sub-habitats within the major ecosystem types mentioned above. Within them, particular life history strategies will allow individual species to proliferate. In upwelling, open water systems, identifying resting forms is still a challenge. Bottom-dwelling resting stages seem to play an important role in semi-confined systems, and may also be important in benthic systems. Advances in the characterization of the life histories and life strategies of benthic HAB species and macrophytes (e.g., Sargassum, Ulva) are also required. Research on the life history of the benthic dinoflagellates genera Gambierdiscus and Ostreopsis is still developing and particular adaptations include flattened shapes and production of mucus to attach to surfaces, which may play a key role in the colonization of surfaces and the development of the blooms. Finally, investigation on the role of toxins as traits that have evolved as a defence mechanism is relatively new (Selander et al. 2015).
Our planet is facing climatic changes at an unprecedented rate and there is an urgent need to assess the potential evolutionary response of HAB species to selective pressures driven by a changing environment. As an example, it has been shown that high CO2 concentration can induce rapid adaptive changes in the genotype composition of toxic cyanobacteria (Sandrini et al., 2016). However, it is important to assess both phenotypic plasticity and genetic adaptation to climate change with appropriate approaches to gain a clear picture (Merilä and Hendry 2014). Cutting-edge -omics technologies will be relevant for advances in these directions. In addition to strain- and species-specific adaptations, advances can be made through generalization of adaptive strategies based on trait-based approaches (i.e., exploring the potential common traits across HAB species) and would facilitate integration of the outcomes from this theme on Adaptive Strategies into new modeling approaches (see Theme 9. Observation, Modeling, Prediction). Furthermore, mixotrophy can constitute a relevant strategy in nutrient acquisition, growth, and production of HAB species, with consequences on the food webs (Mitra et al. 2016).
- Define the characteristics of HAB species that determine their intrinsic potential for growth and persistence.
- Describe and quantify chemical and biological processes affecting species interactions.
- Identify the functional role of cell properties.
- Assess biological traits and intra-specific interactions (e.g., physiology, toxin production, allelochemical properties, life histories, mixotrophy) of HAB species and/or populations in different environments.
- Define and quantify physical-biological interactions at the scale of individual cells.
- Build on the existing knowledge of adaptive strategies of cyanoHABs and macrophyte blooms, including an improved understanding of strain variability and implications for responses to environmental conditions.
- Investigate physical and biological interactions at the scale of individual cells including, for example, grazing selection or avoidance, role of mucus in structuring the benthic environment, active migration and aggregation dynamics, and interactions with bacteria.
- Improve and/or develop observation and analysis systems at the microscale (e.g., micro-cinematography, rheology approaches, imaging-flow cytometry; see also Theme 9. Observation, Modelling and Prediction).
- Determine resting stage formation, deposition and emergence fluxes in the field, with proven methods, and identify the factors affecting the viability and abundance of these life history stages.
- Develop new tools to identify and quantify the various life stages (gametes, zygotes, etc.) and cell growth and mortality rates of HAB taxa in the field.
- Assess the adaptive response of HAB species to environmental change.
Example tasks
- Determine tolerance ranges and optima for growth and toxin production in response to a suite of environmental variables.
- Define the effect of fluctuating environmental regimes on growth processes (reproduction), life history events (excystment and encystment), vertical migration behaviour, nutritional modes (mixotrophy), etc.
- Assess the importance of toxin production (particulate and dissolved fractions, including toxic mucilage) and other cellular properties (cell surface lectin, etc.) in selective grazing.
Outcomes
- Establishment of HAB strains and species within an international network of reference culture collections.
- Identification of key adaptive strategies of particular HAB species.
-
Ecologically based classification of different species according to their adaptations and characteristics.
-
Quantification, parameterization, and prioritization of ecophysiological processes at the cellular level for incorporation into models.
References
The complete list of references can be found here.
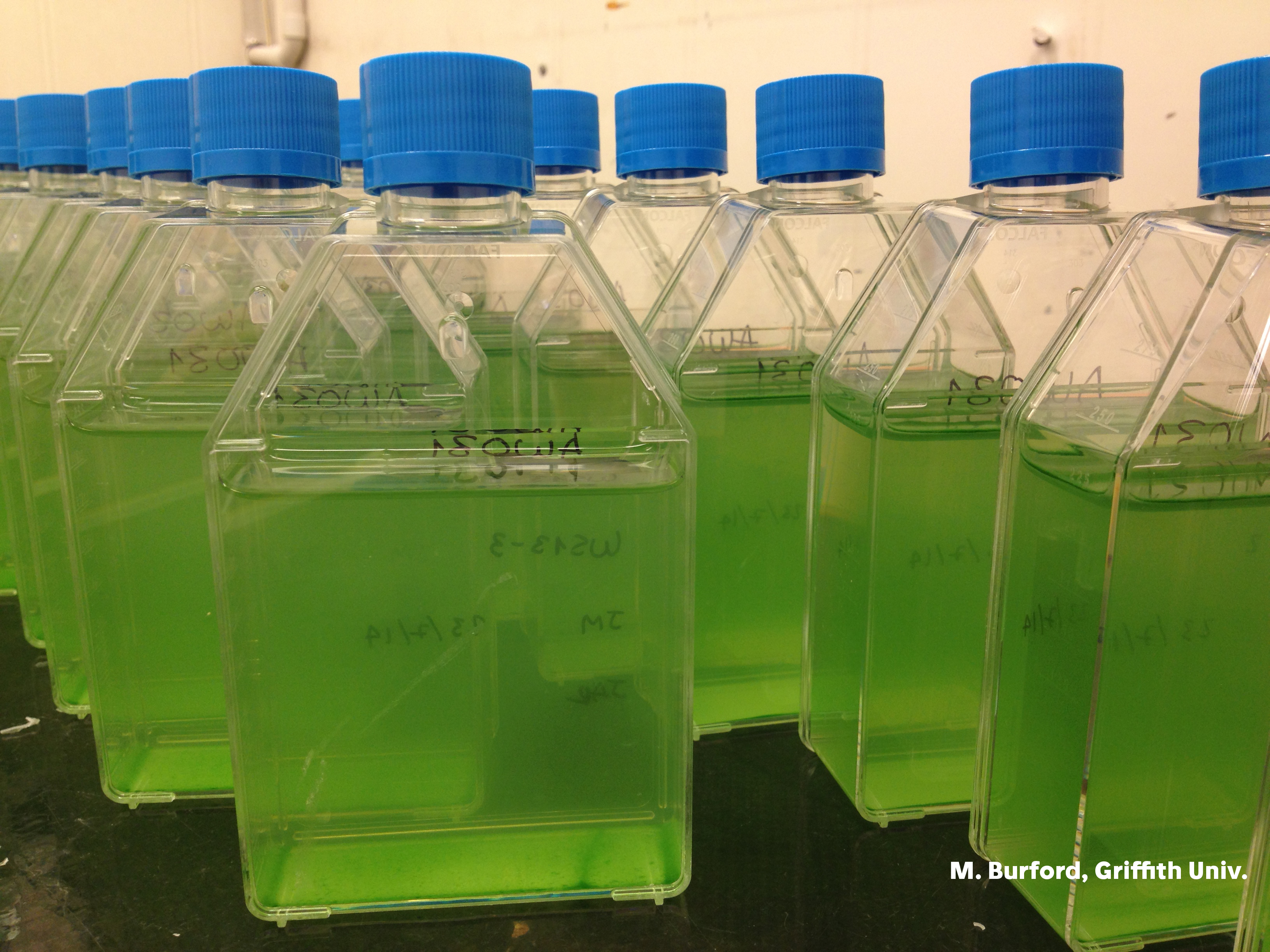
Cultures of cyanobacteria testing the effect of environmental conditions on growth. Photo: Michele Burford.